An Immense World - Ed Yong
- Abhishek Rao
- Aug 13, 2023
- 12 min read
Updated: Mar 23, 2024
Sign Up to receive E-mail updates when a new Blog is posted.

Genre: Science, Nature, Animals, Biology
Rating: 5/5
Date Read: 30/05/2023
Goodreads Profile: Abhishek Rao
Book Link: An Immense World
How do you know but ev’ry Bird that cuts the airy way, is an immense world of delight, clos’d by your senses five? - William Blake
Imagine an elephant in a room. An actual weighty mammal. Imagine the room is spacious enough to accommodate it; make it a school gym. Now imagine a mouse has scurried in, too. A robin hops alongside it. An owl perches on an overhead beam. A bat hangs upside down from the ceiling. A rattlesnake slithers along the floor. A spider has spun a web in a corner. A mosquito buzzes through the air. A bumblebee sits upon a potted sunflower. Finally, in the midst of this, add a human. Let’s call her Rebecca. She’s sighted, curious, and fond of animals. Consider, how Rebecca and the rest of this imaginary menagerie might perceive one another.
The elephant raises its trunk like a periscope, the rattlesnake flicks out its tongue, and the mosquito cuts through the air with its antennae. All three are smelling the space around them, taking in the floating scents. The elephant sniffs nothing of note. The rattlesnake detects the trail of the mouse and coils its body in an ambush. The mosquito smells the alluring carbon dioxide on Rebecca’s breath and the aroma of her skin. It lands on her arm, ready for a meal, but before it can bite, she swats it away—and her slap disturbs the mouse. It squeaks in alarm, at a pitch that is audible to the bat but too high for the elephant to hear. The elephant, meanwhile, unleashes a deep, thunderous rumble too low-pitched for the mouse’s ears or the bat’s but felt by the vibration-sensitive belly of the rattlesnake. Rebecca, who is oblivious to both the ultrasonic mouse squeaks and the infrasonic elephant rumbles, listens instead to the robin, which is singing at frequencies better suited to her ears. But her hearing is too slow to pick out all the complexities that the bird encodes within its tune.
The robin’s chest looks red to Rebecca but not to the elephant, whose eyes are limited to shades of blue and yellow. The bumblebee can’t see red, either, but it is sensitive to the ultraviolet hues that lie beyond the opposite end of the rainbow. The sunflower it sits upon has at its centre an ultraviolet bullseye, which grabs the attention of both the bird and the bee. The bullseye is invisible to Rebecca, who thinks the flower is only yellow. Her eyes are the sharpest in the room; unlike the elephant or the bee, she can spot the small spider sitting upon its web. But she stops seeing much of anything when the lights in the room go out.
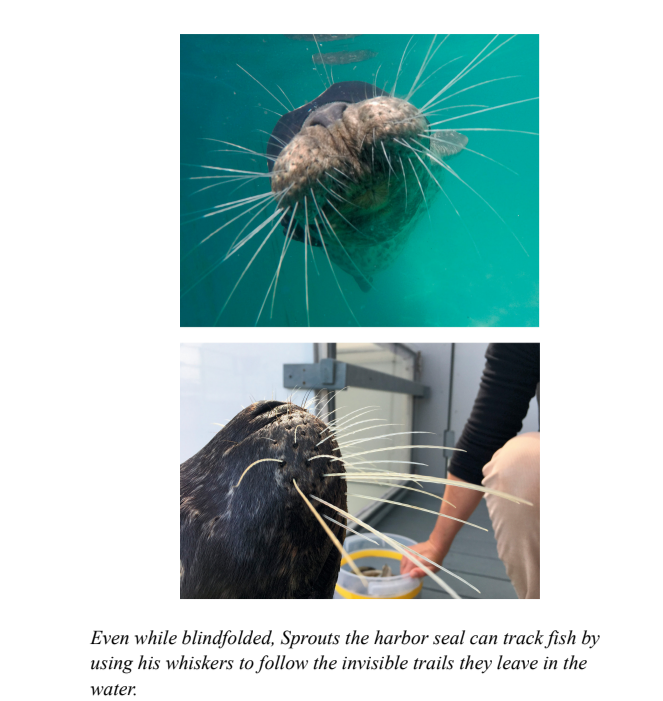
Plunged into darkness, Rebecca walks slowly forward, arms outstretched, hoping to feel obstacles in her way. The mouse does the same but with the whiskers on its face, which it sweeps back and forth several times a second to map its surroundings. As it skitters between Rebecca’s feet, its footsteps are too faint for her to hear, but they are easily audible to the owl perched overhead. The disc of stiff feathers on the owl’s face funnels sounds toward its sensitive ears, one of which is slightly higher than the other. Thanks to this asymmetry, the owl can pinpoint the source of the mouse’s skittering in both the vertical and horizontal planes. It swoops in, just as the mouse blunders within range of the waiting rattlesnake. Using two pits on its snout, the snake can sense the infrared radiation that emanates from warm objects. It effectively sees in heat, and the mouse’s body blazes like a beacon. The snake strikes...and collides with the swooping owl.
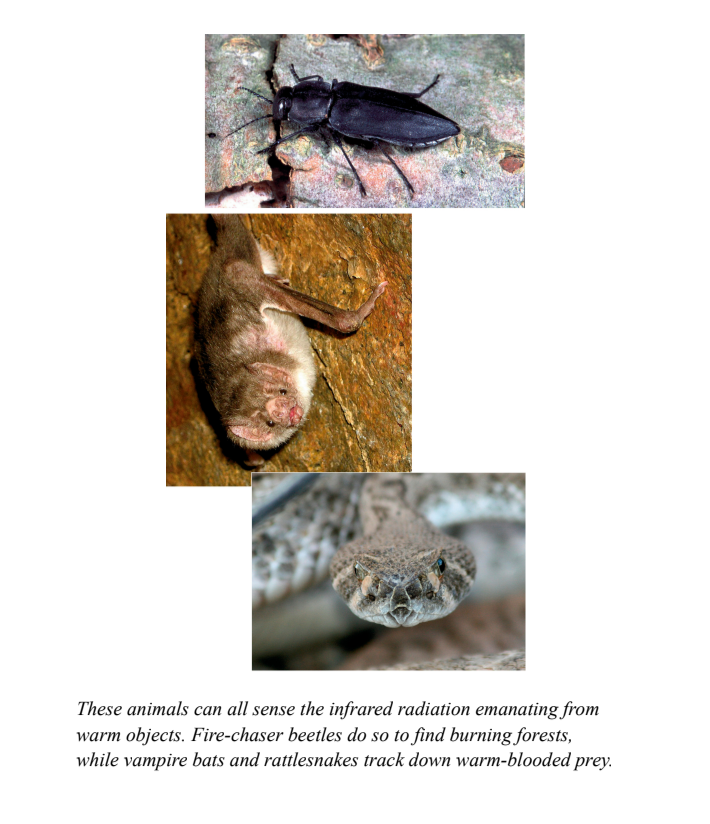
All of this commotion goes unnoticed by the spider, which barely hears or sees the participants. Its world is almost entirely defined by the vibrations coursing through its web—a self-made trap that acts as an extension of its senses. When the mosquito strays into the silken strands, the spider detects the telltale vibrations of struggling prey and moves in for the kill. But as it attacks, it is unaware of the high-frequency sound waves that are hitting its body and bouncing back to the creature that sent them— the bat. The bat’s sonar is so acute that it not only finds the spider in the dark but pinpoints it precisely enough to pluck it from its web.
As the bat feeds, the robin feels a familiar attraction that most of the other animals cannot sense. The days are getting colder, and it is time to migrate to warmer southern climes. Even within the enclosed gym, the robin can feel Earth’s magnetic field, and, guided by its internal compass, it points due south and escapes through a window. It leaves behind one elephant, one bat, one bumblebee, one rattlesnake, one slightly ruffled owl, one extremely fortunate mouse, and one Rebecca. These seven creatures share the same physical space but experience it in wildly and wondrously different ways. The same is true for the billions of other animal species on the planet and the countless individuals within those species. Earth teems with sights and textures, sounds and vibrations, smells and tastes, and electric and magnetic fields. But every animal can only tap into a small fraction of reality’s fullness. Each is enclosed within its own unique sensory bubble, perceiving but a tiny sliver of an immense world.
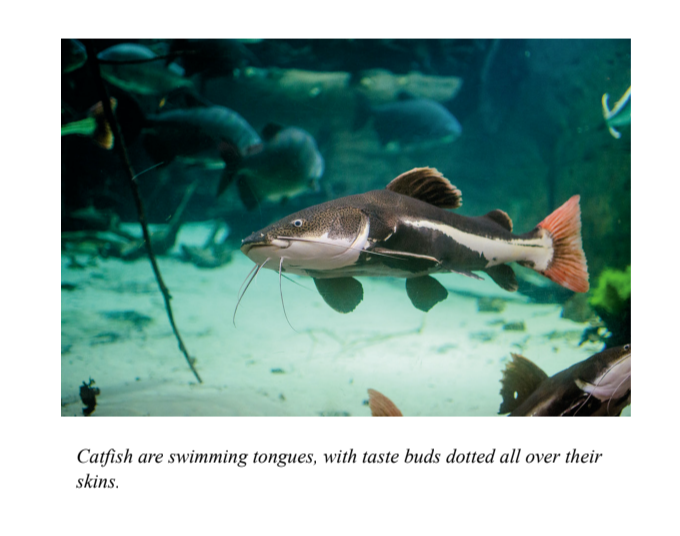
There is a wonderful word for this sensory bubble—Umwelt. It was defined and popularized by the Baltic-German zoologist Jakob von Uexküll in 1909. Umwelt comes from the German word for “environment,” but Uexküll didn’t use it simply to refer to an animal’s surroundings. Instead, an Umwelt is specifically the part of those surroundings that an animal can sense and experience—its perceptual world. Like the occupants of our imaginary room, a multitude of creatures could be standing in the same physical space and have completely different Umwelten. A tick, questing for mammalian blood, cares about body heat, the touch of hair, and the odour of butyric acid that emanates from the skin. These three things constitute its Umwelt. Trees of green, red roses too, skies of blue, and clouds of white are not part of its wonderful world. The tick doesn’t willfully ignore them. It simply cannot sense them and doesn’t know they exist.

In 1879, neuroscientist Paul Broca noted that our olfactory bulbs are relatively puny compared to those of other mammals. He reasoned that smell is a base and animalistic sense, and the loss of it was necessary for us to have higher thought and free will. He then classified us (along with other primates and whales) as non-smellers.
The Jahai people of Malaysia would disagree, as would the Semaq Beri, the Maniq, and the many other hunter-gatherer groups who have dedicated smell vocabularies. The Jahai use a dozen words for smells and smell alone. One describes the scent of gasoline, bat droppings, and millipedes. Another is for some quality shared by shrimp paste, rubber tree sap, tigers, and rotten meat. Yet another refers to soap, the pungent durian fruit, and the popcorn-like twang of the binturong. They “have this ease of talking about smells,” says psychologist Asifa Majid, who found that the Jahai can name smells as easily as English speakers can name colours. Just as tomatoes are red, the binturong is ltpit.
Elephants that have returned to postwar Angola seem to skirt around the millions of landmines that still dot the land—unsurprising, perhaps, given how quickly they can be trained to detect TNT.
Since Aristotle, scholars believed that vultures had a keen sense of smell. Audubon thought differently. When he left a putrefying pig carcass in the open, no vultures came to eat. By contrast, when he put out a deerskin stuffed with straw, a turkey vulture swooped in and pecked away. These birds, he claimed in 1826, find their food with sight, not smell. His supporters bolstered that claim with equally dodgy evidence. One noted that vultures would attack a painting of an eviscerated sheep and that captive vultures refused to eat after being blinded. Another showed that a turkey - not a turkey vulture; an actual turkey - would still eat food that was tainted with sulfuric acid and potassium cyanide, a strong-smelling concoction that proved violently fatal.
Never mind that vultures prefer fresh carcasses and ignore overly stinky meat like the kind Audubon used. Forget that Audubon confused black vultures (less reliant on smell) with turkey vultures, or that oil paints at the time gave off certain chemicals also found in decaying flesh. Disregard the many reasons a mutilated animal might not feel very peckish. The idea that turkey vultures—and by dubious extension, all birds - can’t smell became textbook wisdom. Evidence to the contrary was ignored for decades, and the study of avian olfaction lapsed into neglect.

Look inside the eyes of a jumping spider, a human, or any other animal, and you’ll find light-detecting cells called photoreceptors. These cells might vary dramatically from one species to another, but they share a universal feature: They contain proteins called opsins. Every animal that sees does so with opsins, which work by tightly embracing a partner molecule called a chromophore, usually derived from vitamin A. The chromophore can absorb the energy from a single photon of light. When it does, it instantly snaps into a different shape, and its contortions force its opsin partner to reshape itself, too. The opsin’s transformation then sets off a chemical chain reaction that ends with an electrical signal travelling down a neuron. This is how light is sensed. Think of the chromophore as a car key and the opsin as an ignition switch. The two fit together, light turns the key, and the engine of vision whirs into life.
The biologist Dan-Eric Nilsson says that eyes evolve through four stages of increasing complexity.
The first just involves photoreceptors - cells that do little more than detect the presence of light. The Hydra, a relative of jellyfish, uses photoreceptors to ensure that its stings fire more readily in dim light perhaps it does this to save those stings for nighttime hours, when its prey is more common, or to deploy them when it senses the shadow of a passing target.
Olive sea snakes have photoreceptors at the tips of their tails, which they will pull away from sources of light. Octopuses, cuttlefish, and other cephalopods have photoreceptors dotted throughout their skin, which might help to control their amazing colour-changing abilities.
In the second stage, photoreceptors gain shade—a dark pigment or some other barrier that blocks the light coming in from certain angles. Shaded photoreceptors can detect light’s presence and infer its direction. These structures are still so simple that many scientists don’t even regard them as genuine eyes, but they are useful to their owners nonetheless. They can also show up anywhere.
The Japanese yellow swallowtail butterfly has photoreceptors on its genitals. A male uses these cells to guide his penis over a female’s vagina, and a female uses them to position her egg-laying tube over the surface of a plant.
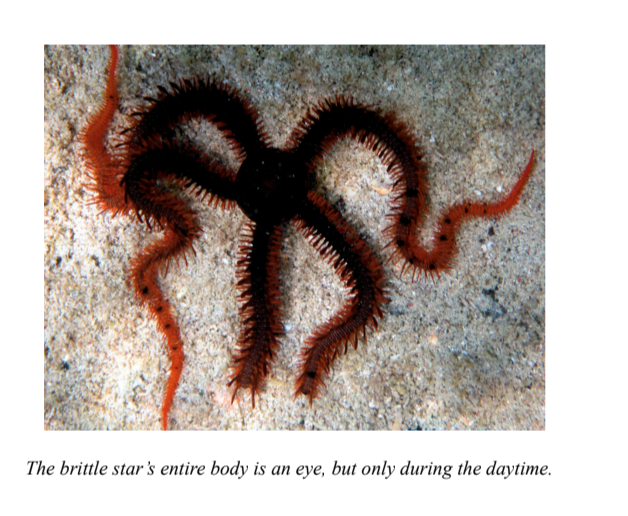
In the third of Nilsson’s stages, shaded photoreceptors cluster into groups. Their owners can now knit together information about light from different directions to produce images of the world around them. For many scientists, this is the point when light detection becomes an actual vision when simple photoreceptors become bona fide eyes, and when animals can truly be said to see.
At first, their vision is blurry and grainy, suitable only for crude tasks like finding shelter or spotting looming shapes. But with the addition of focusing elements like lenses, their view sharpens, and their Umwelt fills with rich visual detail.

High-resolution vision is the fourth of Nilsson’s stages. When it first appeared, it would have intensified the interactions between animals. Conflicts and courtships could play out over distances longer than touch or taste would allow and at speeds too fast for the smell. Predators could now spot their prey from afar, and vice versa. Chases ensued. Animals became bigger, faster, and more mobile. Defensive armour, spines, and shells evolved.
The rise of high-resolution vision might explain why, around 541 million years ago, the animal kingdom dramatically diversified, giving rise to the major groups that exist today. This flurry of evolutionary innovation is called the Cambrian explosion, and stage-four eyes might have been one of the sparks that ignited it.
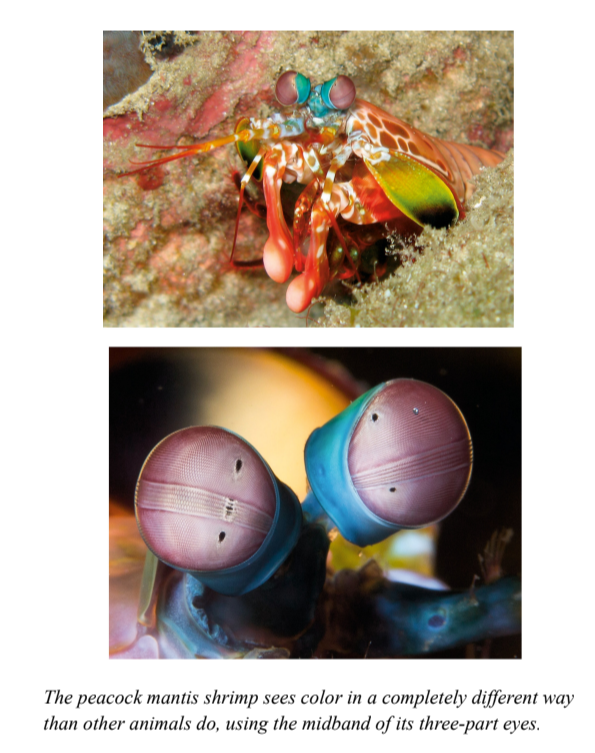

Nilsson’s four-stage model addresses a concern of Charles Darwin, who was unsure how complex modern eyes could have evolved. “To suppose that the eye, with all its inimitable contrivances...could have been formed by natural selection, seems, I freely confess, absurd in the highest possible degree,” he wrote in The Origin of Species. “Yet reason tells me, that if numerous gradations from a perfect and complex eye to one very imperfect and simple, each grade being useful to its possessor, can be shown to exist...then the difficulty of believing that a perfect and complex eye could be formed by natural selection, though insuperable by our imagination, can hardly be considered real."
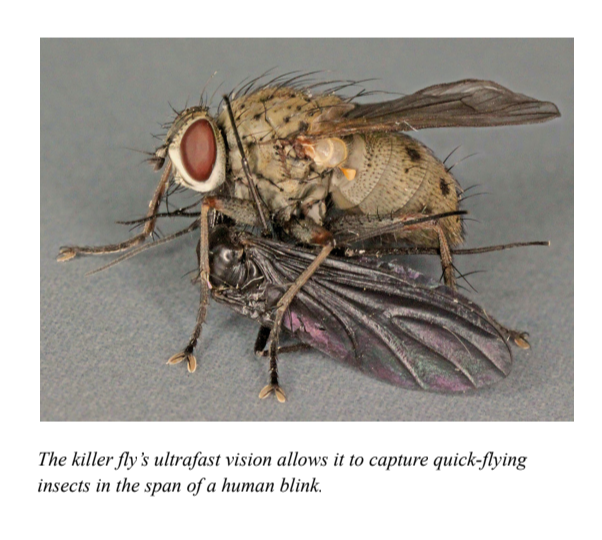
A starfish has eyes on the tips of its five arms. These eyes can’t see colour, detail, or fast movements, but they don’t have to. They only have to detect large objects, so that the starfish can slowly amble back toward the safety of a coral reef. A starfish has no need for an eagle’s acute eye, or even a jumping spider’s. It sees what it needs to. The first step to understanding another animal’s Umwelt is to understand what it uses its senses for.
Humans outshine almost every other animal at resolving detail. Our exceptionally sharp vision gives us a rarefied view of a zebra’s stripes. On a bright day, people with excellent eyesight can distinguish the black-and-white bands from 200 yards away. Lions can only do so at 90 yards and hyenas at 50 yards.
Look at a full, living scallop, and you’ll see a very different animal. And that animal will see you, too. Each half of a scallop’s fan-shaped shell has eyes arrayed along its inner edge—dozens in some species, and up to 200 in others. In the bay scallop, the eyes look like neon blueberries.

A small species of hammerhead can detect an electric field of just one nanovolt—a billionth of a volt—across a centimetre of water. A shark’s electric sense only works at short range, however. It can’t sense a buried fish (or electrode) from across an ocean, or even from across a pool. It has to be within arm’s length of its target. Over mile distances, a shark sniffs out its food. As it draws near, vision takes over. Nearer still, the lateral line (senses vibrations in the water) chips in. Its electric sense only enters the fray at the close of the hunt, to pinpoint the exact position of its prey and guide its strike. That’s why the ampullae of Lorenzini are usually concentrated around the mouth.


Imagine, what it might be like to be a mosquito. Flying through a thick soup of tropical air, your antennae slice through plumes of odorants until they catch a whiff of carbon dioxide. Enticed, you turn into the plume, zigzagging when you lose track of it, and surging ahead whenever you pick it up. You spot a dark silhouette and fly over to investigate. You enter into a cloud of lactic acid, ammonia, and sulcatone—molecules released by human skin. Finally, the clincher: an alluring burst of heat. You land, and your feet pick up an explosion of salt, lipids, and other tastes. Your senses, working together, have once again found a human. You find a blood vessel and drink your fill.
When animals move, their sense organs provide two kinds of information. There’s exafference, signals produced by stuff happening in the world. There’s also reafference, signals produced by an animal’s own actions. I still struggle to remember the difference between these, and if you share that problem, you can think of them as other-produced and self-produced.
From my desk, I can see the branches of a tree rustling in the wind. That’s exafference—other-produced. But to see those branches, I had to look to my left—a sudden, jarring movement that sent patterns of light sweeping across my retinas. That’s reafference—self-produced. Every animal has to distinguish between these two kinds of signals for each of its senses. But here’s the catch: These signals are the same from the point of view of the sense organs.
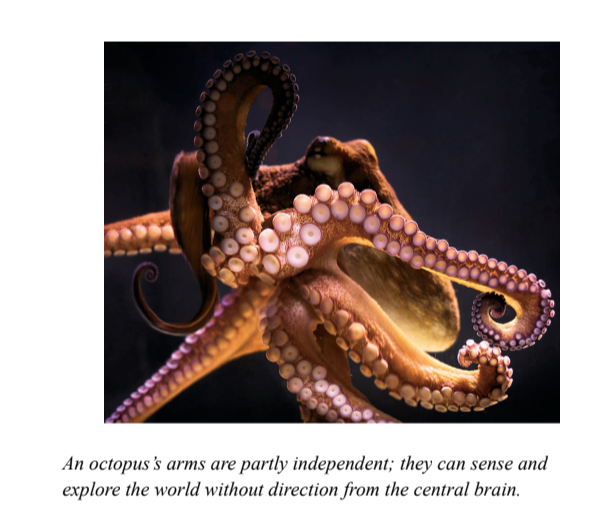
An octopus’s central nervous system contains around 500 million neurons—a total that dwarfs that of all other invertebrates and that’s comparable to the number found in small mammals. But only a third of these neurons are located in the animal’s head, within the central brain and the adjacent optic lobes that receive information from the eyes. The remaining 320 million are in the arms. Each arm “has a large and relatively complete nervous system, which seems barely to communicate with the other arms,”
Even the 300 suckers on each arm are somewhat independent. Once a sucker makes contact with something, it reshapes itself to create a seal and then sticks by creating suction. Meanwhile, it simultaneously touches and tastes using 10,000 mechanoreceptors and chemoreceptors on its rim. Our tongues perceive flavour and mouthfeel as separate qualities, but given the wiring of the sucker, an octopus likely doesn’t. Its sensations of taste and touch “are probably inextricably fused” in a way that resembles synesthesia.
Depending on the flavors it feels, or the textures it tastes, the sucker might continue sucking or let go. And it can make that decision independently since each sucker is served by its own mini-brain—a dedicated cluster of neurons called the sucker ganglion. The suckers’ independence is obvious when watching disembodied arms, which are often found stuck to the sides of fish but will never stick to other arms from the same octopus.
The central brain can control the arms, but it’s a relaxed boss. It doesn’t like to micromanage but coordinates its team of eight when needed. A single arm can snake its way through an opaque maze, using taste touch to find the right route with no input from the rest of the animal. But it has been shown that octopuses can also solve problems that stump individual arms.
They set up a transparent maze in which the correct path forced the arm out of the water, depriving it of chemical cues. The octopuses could still find that path by guiding their arms with their eyes, but it didn’t come naturally to them. It took a while for them to learn how to do it, and one individual out of seven never did.
Amazon Link (India): An Immense World
Amazon Link (International): An Immense World
As an Amazon Associate, I earn from qualifying purchases.
Like, Share and Comment
Sign Up to receive E-mail updates when a new Blog is posted.
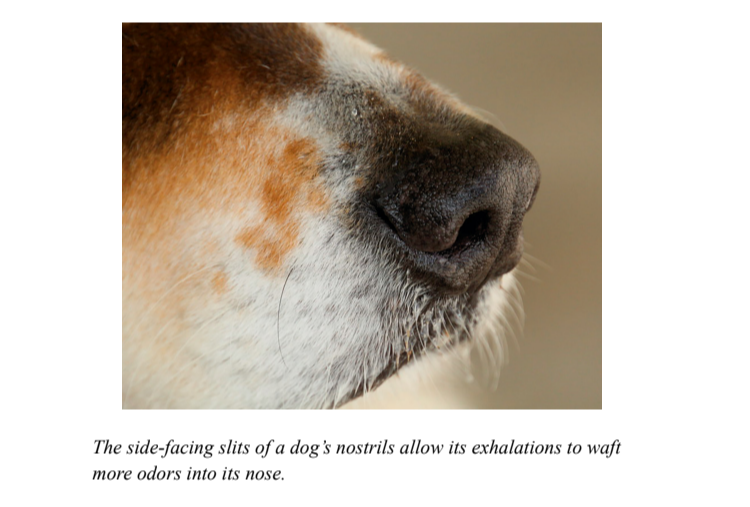
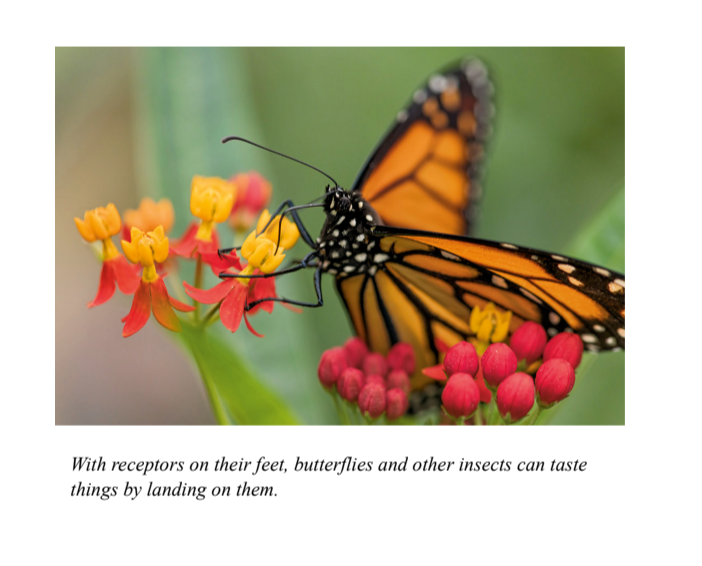
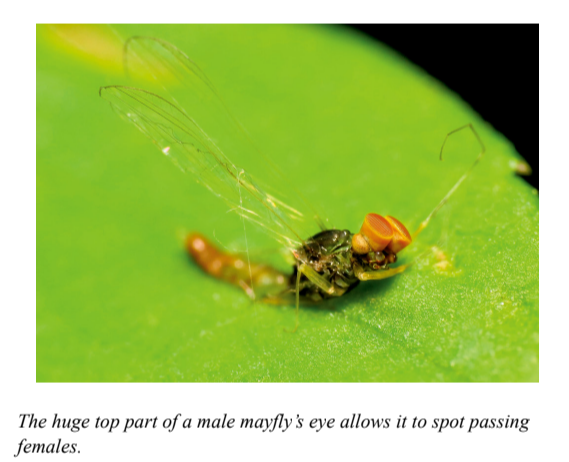

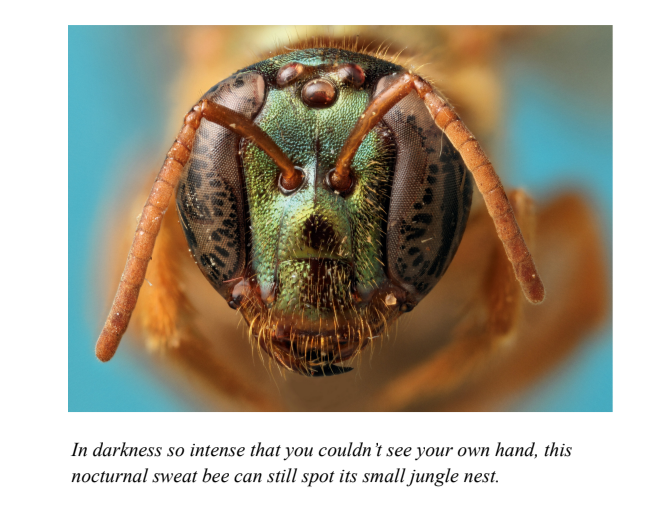
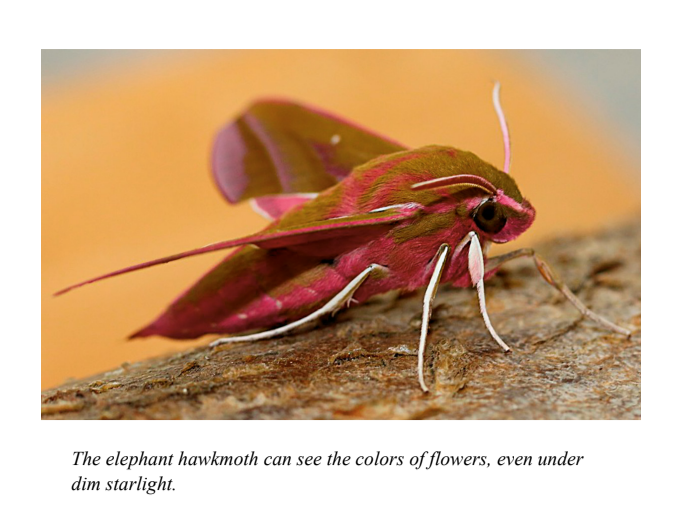
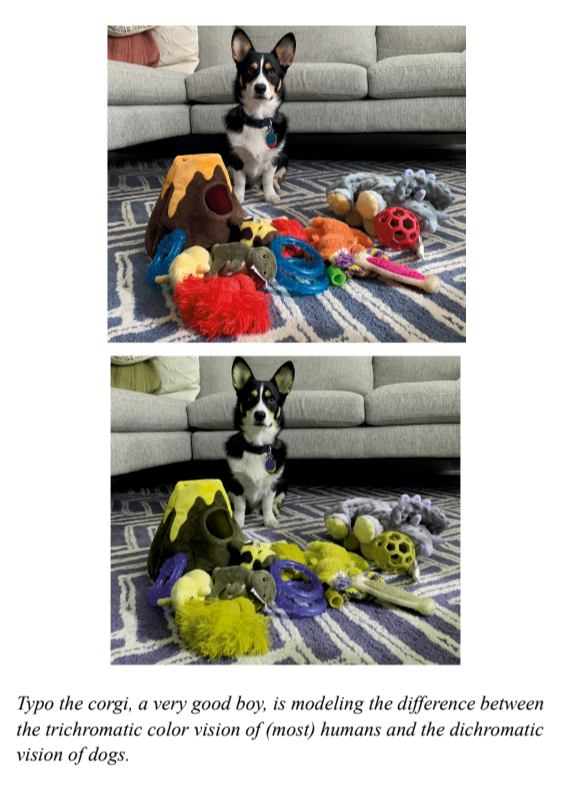
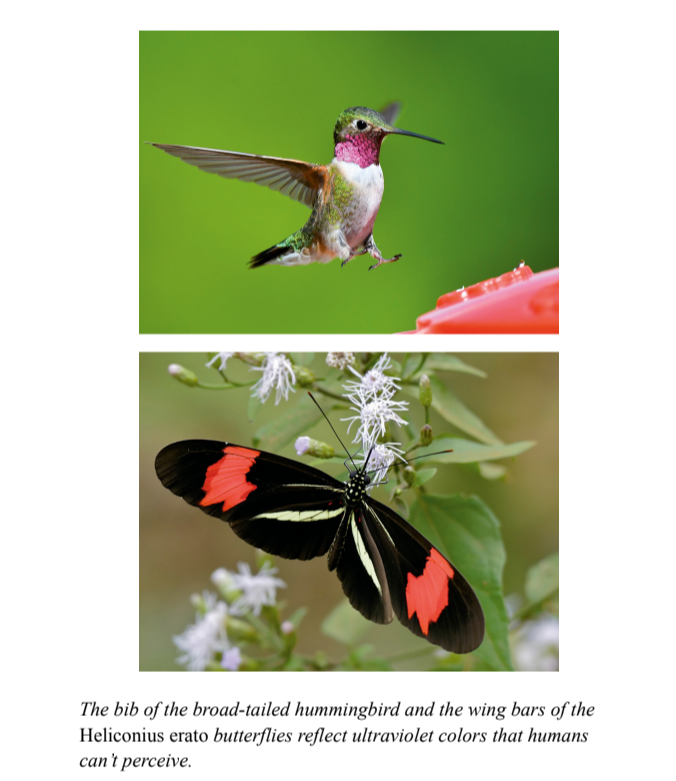
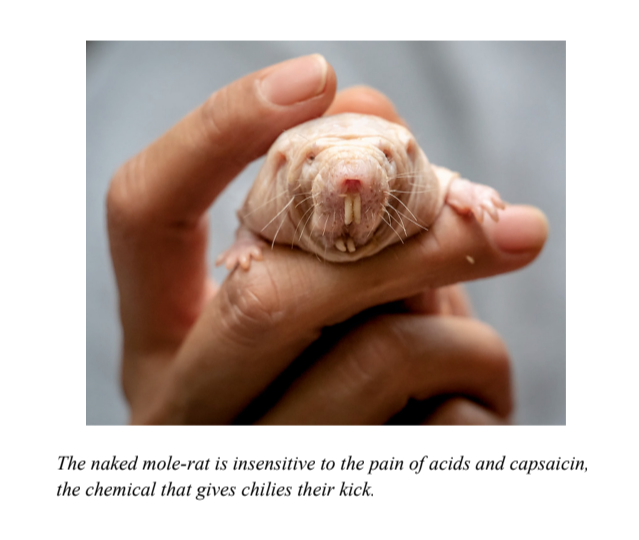
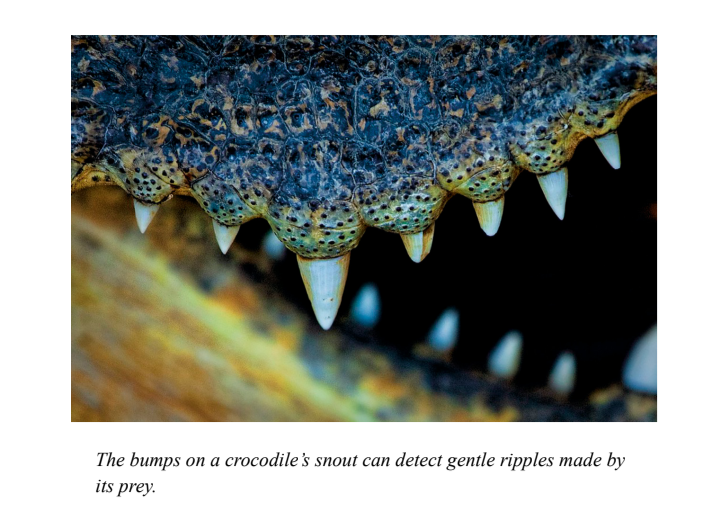
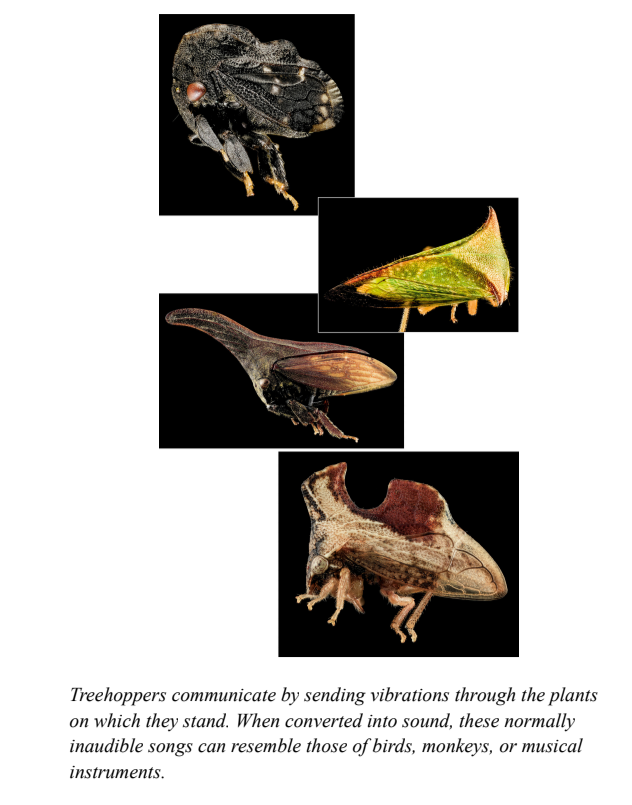
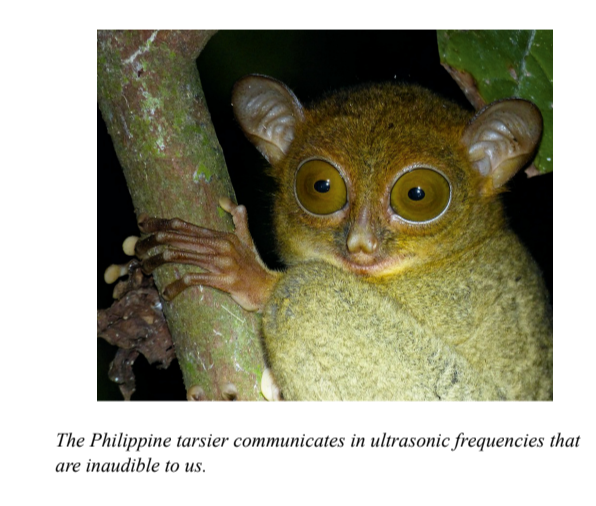
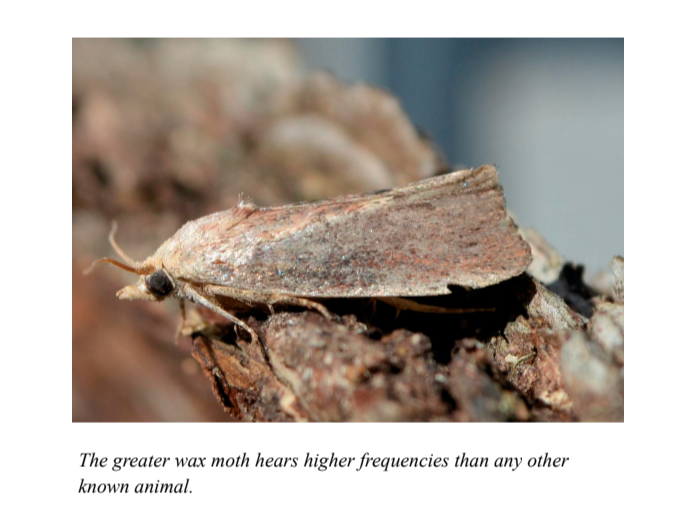
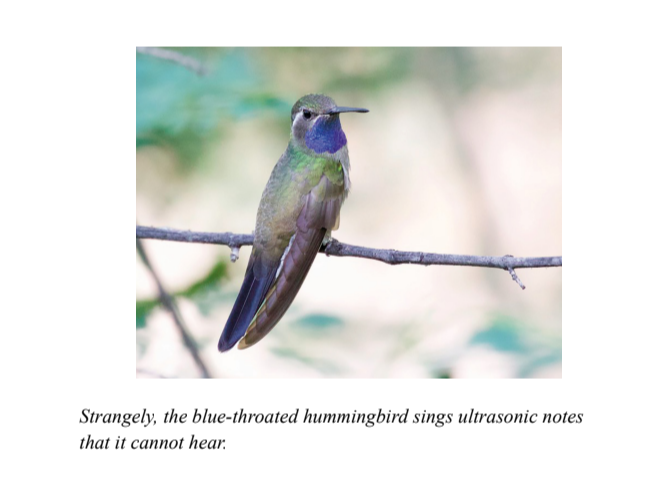


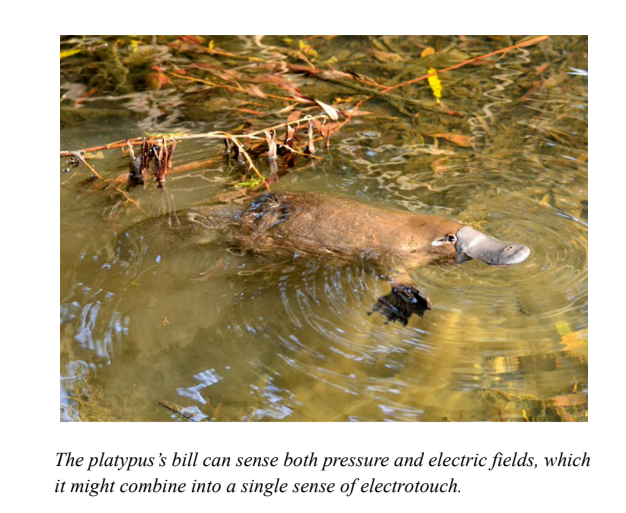
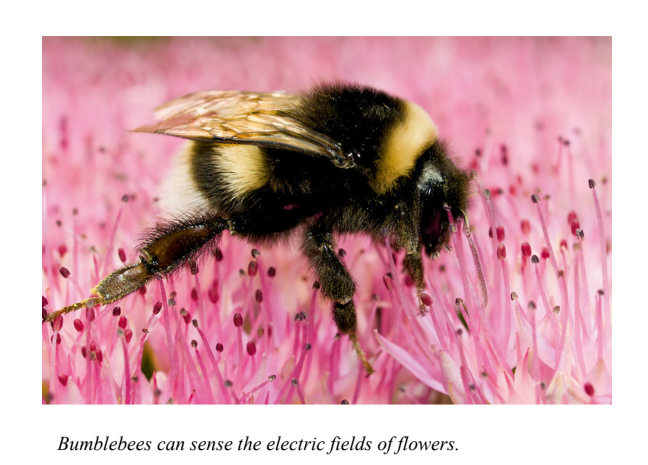

Book Link: An Immense World
Like, Share and Comment
Sign Up to receive E-mail updates when a new Blog is posted.
Comments